Main Page: Difference between revisions
→How to escape from Earth?: picture |
→News: SpaceUp |
||
(52 intermediate revisions by the same user not shown) | |||
Line 1: | Line 1: | ||
=N-Prize reflections= | =N-Prize and reflections on low-cost access to space= | ||
This | This Web site aims to gather research in the field of astronautics, rocketry and other technologies that can be used for the N-Prize competition, and more generally, to put microsatellites in orbit at low cost. It is not an official Web site for the [[N-Prize]], the official being here: http://www.n-prize.com/. The goal of this competition is roughly to reproduce the great achievement of the Sputnik in 1957, but for a 20g satellite and with spending less than £1000. However, this Web site and its associated research will not stop after the contest is over, this is more a long term (should I say lifetime?) project. It is hosted by the Open Technology And Science Knowledge Initiative ([http://otaski.org OTASKI]). | ||
I'm not part of a team for the N-Prize, nor did I register one, because I don't really have the expertise and resources to actually build something in time before the deadline of the contest in september 2013. Anyway, if you find this project interesting, [[Join|join]] and [[Guidelines|participate]]! Maybe if we are enough to work on the project, it is possible to make it in time. It is also possible to provide a part of the challenge and join together with another team providing the other part. Other teams have for example been developing satellites, rocket engines, and so on. | |||
==What is the LCAS project?== | |||
LCAS, standing for low-cost access to space, aims to provide an '''open and innovative low-cost orbital launch system for very small satellites''' (less than 10 kg). Research has led us to consider using an aircraft for rocket launches (air-to-orbit), the body of the plane being the rocket itself. The rocket, or a part of it, as in any other orbital launch system, would achieve orbit and thus could embed a minimum of science, making optional the use of a real satellite as payload. Since the main constraint is to have low costs, we'll have to design and build the carrier plane first, including its turbofan engines, which is probably the hardest part of the whole project, and as far as we know has never been done by amateurs. | |||
We thus currently focus on the turbofan [[Build_a_cheap_turbofan|research and design]], on which depends everything else. We may then consider helping other N-Prize teams if this is done in time, or other similar projects outside the contest, by providing them those engines and help with aircraft design and rocket integration. Some other parts of the aircraft/rocket are also being studied, for example the [[EmbeddedRocketComputer|software control]] and the low-cost [[EmbeddedRocketComputer#Sensors|sensors]] that can be used to render the aircraft autonomous at first, then make the rocket go into space and reach a controlled orbit. | |||
==News== | |||
''News are also available on twitter [https://twitter.com/OTASKI @OTASKI]'' | |||
'''''October 2017:''''' A talk was made at SpaceUp about the use of nano-spacecraft as a cheap way to explore the solar system (spoiler: there is no cheap way). See the [[:File:Solar_system_exploration_with_cubesats.pdf|presentation]]. | |||
'''''December 2015:''''' CFD is has resumed for a few months. Basic [[Telemetry|RF telemetry]] has been validated using a high-altitude balloon, thanks to the resources of [https://ukhas.co.uk/ ukhas], but for a rocket, a much more important data rate is required. That would either require more directional receiving antennas or a more powerful emitter, which requires an amateur radio license. | |||
Most pages on this wiki are outdated or unfinished and would not be useful for somebody familiar with aerospace. There is one page that can be still useful, about [[Rocket:First_approximations|rocket mass estimation]]. A simple program was developed and some graphs are shown at the bottom, to easily compute and visualise which parameters cause the mass of an air-to-orbit rocket to change. | |||
'''''December 2013:''''' CFD has been stalled for a few months. In parallel, work on [[Telemetry|RF telemetry]] has started. First prototype will be tested in a high-altitude balloon in August 2014, in order to validate the RF module and the ground receiver. | |||
'''''March 2013:''''' Since December, learning CFD has been the main activity, and it will probably remain so next months. [[CFD:Introduction|A page]] has been created to give CFD beginners some interesting links. CFD has a steep learning curve, but learning how to use it will have huge benefits for the project in the long term: | |||
* evaluate the lift and drag associated with supersonic wings/fins on the aircraft-launched rocket will enable us to refine the rocket mass ([[File:Rocket_mass.c]]) model | |||
* hopefully have a theoretical validation of our high-altitude turbofan [[Turbofan:Alternative_Designs|alternative design]] | |||
* simulate different wing profiles at high-altitude subsonic conditions for the carrier aircraft | |||
* evaluate aircraft and engine capability on lift-off and early flight conditions while they are both tailored for high altitude flight | |||
* refine the heat transfer approximations ([[File:Heat_transfer_to_rocket_tank.c]]) for cryogenics tank vaporization, used in the rocket mass model for tank dimensioning, with a better climb profile input too. | |||
'''''December 2012:''''' A simple rocket trajectory model has been made in order to evaluate the trajectory of an aircraft- or balloon-released-rocket. It appears that the Delta V taken for granted for gravity drag for these rockets, around 800 m/s, is quite erroneous, or not possible with the expected overall thrust-to-weight ratio. Without taking into account the aerodynamic effects like lift and drag, for a ratio of around 1.5, the Delta V for gravity drag is at least 1300 m/s. To reduce it, the ratio should be higher, like 3.0, in that case it may be possible to have only 800 m/s Delta V for gravity, but the mass of the engine would be much higher. See [[Rocket:First_approximations#The_gravity_drag_and_overall_thrust-to-weight_ratio_issue|a solution]] to this issue. | |||
The program evaluating the mass of the rocket has been refined (v3), and the forgotten pipe linking the upper tank to the engine adds approximately 150 kg of wet mass too ([[File:Rocket_mass.c]]). | |||
Incoming works will study aerodynamic lift and drag of a simple rocket and of a rocket with small supersonic wings to better evaluate the gravity drag for our mass evaluation. | |||
'''''November 2012:''''' Rocket mass [[Rocket:First_approximations#Our_first_approximation|has been estimated]] to 150 kg. Turbofan engines parameters can now be calculated for a real application: aircraft carrier for air-to-orbit single stage rocket, tailored for pico and [https://en.wikipedia.org/wiki/Miniaturized_satellite#Nanosatellite nanosatellites]. | |||
Also, [[Rocket:First_approximations#Effects_of_parameter_changes|graphs]] have been created to illustrate the importance of various rocket design parameters, such as thrust-to-weight ratios, mass ratio, payload mass. The program ([[File:Rocket_mass.c]]) modelling the mass of rockets is being refined and the cryogenic propellant vaporization has been evaluated by another program ([[File:Heat_transfer_to_rocket_tank.c]]). Both programs are freely available. | |||
'''''August 2012:''''' A first step in the project realization will be a turbofan's compressor blade manufacturing, in order to validate the manufacturing process suitability and low cost for the turbofan. The first compressor stage prototype has to be designed in this optics. However, that requires having a [[Rocket:First_approximations|first approximation]] of the rocket mass in order to also have an estimation of the aircraft size and mass, from which we can estimate turbofan engine's properties: inlet speed, required thrust, blade length, RPM and so on. Blade manufacturing will mostly rely on a thermocaster that we'll have to design too. | |||
'''''May 21, 2012:''''' Boeing [http://www.aviationweek.com/Article.aspx?id=/article-xml/AW_05_21_2012_p25-458597.xml has also announced] its low cost orbital launch system, based on the WhiteKnightTwo carrier craft and a hypersonic air-breathing first and second stages. | |||
'''''May 2012:''''' Study of aerodynamics is still heavily under way in order to validate our [[Turbofan:Alternative_Designs|alternate turbofan mode of operation]]. This is the first thing to validate before the project can enter a real engine design phase of the engine, which will in turn allow the plane to be designed. | |||
==How to escape from Earth?== | ==How to escape from Earth?== | ||
Rockets have been used for more than 50 years to escape the gravity of earth. They are good for three things: create an important thrust, go fast, and burn a large amount of propellant. Indeed, the efficiency of a propulsion engine is measured with specific impulse (''I<sub>sp</sub>''), and for rocket engines, it is quite low. However, their engine is the only engine that provide the sufficient thrust to climb up with large speeds and to tear of Earth's gravity. | |||
Besides altitude, speed is the most important factor when trying to put an object into orbit. Without it, satellites would fall back down on Earth, even if you climb up at 200 miles. Once again, rocket engines, with their high thrust power can achieve sufficient speed (> 8 km/s) before falling back on Earth. | |||
Rocket trajectories generally tend to form a curve nearing the square angle, with the beginning of the flight being orthogonal to Earth and the final direction being parallel to Earth's surface. The reason is that since they achieve ultrasonic speeds very quickly, the dynamic air pressure on their body (mainly the fairing), resulting in drag, becomes quite important. It is more efficient to first escape the low atmosphere, with its 85% of its whole mass below 11km altitude, and then pitch to gain the horizontal speed needed for orbital injection without being slowed down by atmospheric friction. | |||
Rocket | [[Image:Rocket_trajectory.png|center|Rocket trajectory: initial vector is vertical, final is tangent]] | ||
That particular point of the cost of escaping the atmosphere made me thought about using an aircraft to launch a rocket from the upper atmosphere, reducing considerably the air pressure, the drag, and improving trajectory and efficiency. Moreover, the specific impulse of a turbofan is around ten times greater than the Isp of a rocket engine, since it uses oxygen from the atmosphere to burn its fuel, and not some on-board oxidizer. See [http://gravityloss.wordpress.com/2008/04/21/air-breathers-advantage this article] for more information on the differences of rocket and aircraft propulsion efficiency. For the N-Prize, the cost of the aircraft could be deducted from the overall price since if it can be reused. | |||
I started searching and I found out that Orbital already has developped an [https://en.wikipedia.org/wiki/Air_launch_to_orbit air-to-orbit] launch vehicle, called the [https://www.orbital.com/SpaceLaunch/Pegasus/ Pegasus]. It is able to push onto Low Earth Orbit a payload up to 1,000 lbs (450 kg), and it is launched from a full-sized airplane. My goal is thus to study the feasibility of something similar, at very low price, even for the aircraft. A rocket would still be used for air-to-orbit link because nothing else is able to achieve a speed around 9 km/s before falling back on Earth. Some specific technologies can be used to improve efficiency, as explained below in the [[#The rocket|rocket]] section. | |||
Several N-Prize teams are working on using Helium or Hydrogen balloons ([https://en.wikipedia.org/wiki/Rockoon rockoons]) to get to the high atmosphere, up to 35 or 40 km and then launch a rocket. It is a nice solution too, and maybe less expensive in the overall, but balloons are not reusable, suffer from imprecise trajectory due to winds, and provide no initial speed. The initial speed of an aircraft carrier would be quite low too in our first designs, but the potential for a supersonic velocity release is not shut. | |||
Single stage to orbit (SSTO) are also a promising research field for low-cost orbiting. In [http://www.youtube.com/watch?v=esgc5W_Ufng this video] (SpaceX guys), here captured at SpaceUP, they don't even predict the use of attitude control outside the atmosphere to avoid expensive guidance actuators. The main idea of SSTO is that the launch system (rocket) ''is'' the payload. It does not even aim to insert a smaller satellite into orbit. | |||
==The aircraft== | ==The aircraft== | ||
Some aircraft have been exploring the high atmosphere, around 30km high. Contrary to what one would assume, high flight speeds are not needed, if the weight is kept low. The [http://www.nasa.gov/centers/dryden/news/ResearchUpdate/Helios/index.html Helios] for example, autonomous solar powered aircraft, flights at this altitude at 20km/h. John Powell ([http://www.jpaerospace.com/ JP Aeroospace]) is also researching on high altitude propellers and plans to make it to space using a high altitude base for payload transfer to a bigger plane. He describes it well in this [http://www.youtube.com/watch?v=G7LQuVfDwFQ video] interview. The [https://en.wikipedia.org/wiki/Lockheed_U-2 U-2] is a manned reconnaissance aircraft flying at 21km altitude, cruising at relatively high speeds (690km/h). Those planes are designed with a very long wingspan, and low weight, similar to gliders. | |||
Another kind of design is the fighter jet, for example the [https://en.wikipedia.org/wiki/Mig_25 MiG-25] which also was an altitude (amongst other) record breaker. It had two powerful turbojet engines with afterburner, allowing him to reach a service altitude of 20km and a maximum altitude of more than 37km. It however required a thrust (200kN) equivalent to the empty weight of the plane and large amounts of fuel to climb this high. The same is true for the [https://en.wikipedia.org/wiki/Sr-71 SR-71]. | |||
These concerns of how high altitude is reached - mainly through high engine power or high lift at subsonic flight - is discussed on the page dedicated to [[Flight at high altitude|high altitude flight]]. Currently, the subsonic way is being studied, both for rocket and the aircraft, since a higher release velocity means that the rocket can be smaller, and consequently the plane too. | |||
Nevertheless, we would benefit from speed of the aircraft, speed that wouldn't be needed by the rocket to reach. It is a low speed compared to orbital speed though. Supersonic launch speed would be nice, but very hard to achieve. Currently, only subsonic speed is considered in the project. | |||
The major issue with the aircraft is [[Build a cheap turbofan|how to build a £100 turbofan?]] Small turbofan engines exist, but are made for or by the military, so very expensive, very reliable, and their use is restricted to missiles or UAVs. Small gas turbine engines exist however, even in an axial design, see [http://www.bladonjets.com/technology/gas-turbines/ Bladon Jets] or [http://www.price-induction.com/en/dgen/caracteristiques/ Price Induction] for a commercial turbofan for very small jets (nice picture of the fan [http://www.price-induction.com/site_media/images/dgen-net/technologies/optimisation_de_masse_grand.jpg here]). | |||
Links on wing or aircraft design related to speed and altitude: [http://forum.avsim.net/topic/328556-u-2-climb-rate/page__st__50#entry1952184]. | |||
===Staging and recovery=== | |||
''Main page for ground-related aircraft operation and return trip: [[Aircraft Mission]].'' | |||
Separation from the aircraft is a big concern. If wings and tail are directly mounted on | |||
Separation from the | |||
the rocket body and jettisoned, they would not need some guidance or attitude | the rocket body and jettisoned, they would not need some guidance or attitude | ||
control electronics, just a basic parachute system. If the wings are able to fly | control electronics, just a basic parachute system. If the wings are able to fly | ||
without the rocket, two guidance systems are needed: one for the rocket and one | without the rocket, two guidance systems are needed: one for the rocket and one | ||
to get back the aircraft in one piece for future launches. | to get back the aircraft in one piece for future launches. Keeping the N-Prize in mind, the aircraft part of the space launch system should be reusable, so that it doesn't count in the £1000 limit. In that case, it has to be recovered in good condition, either using a chute and a GPS tracker, or a more complicated autonomous or remotely operated return-to-runway and landing system. | ||
===Guidance=== | ===Guidance=== | ||
''Main page: [[EmbeddedRocketComputer|embedded computer]].'' | |||
A satellite navigation system may be used in the plane for position tracking if allowed by their owning entities. Other sensors should be shared with the rocket's embedded computer, if choices made for staging and recovery allow it. | |||
Sun position can be a very good and easy indicator of attitude, as well as earth curve recognition. Video camera is likely to be the main sensor, since it can provide lots of information for very low cost (but for high processing power). | |||
==The rocket== | ==The rocket== | ||
''Main page: [[RocketEngines|rocket engines]], Category page: [[:Category:Rocket|list of pages]].'' | |||
Some concerns are emphasized in this section, some choices are made too. A list of concerns and how they are handled by existing engine designs can be found on the [[RocketEngines|rocket engines]] page. For the first approximations of the capabilities and properties of our rocket and rocket engine, for example the minimum weight required to achieve orbit, see the [[Rocket:First_approximations|first approximations]] page. | |||
===Fuel=== | ===Fuel=== | ||
''Main page: [[Propellants]].'' | |||
Propellants represent the most important part of the weight of what we have to launch. It should thus be chosen carefully regarding to its cost, but also regarding their efficiency, the Isp they can produce. This is [[Rocket:First_approximations#Effects_of_parameter_changes|especially important]] in an SSTO design. | |||
Alcohol has been used in the early ages of rocketry, in the German V-2 for example. It has the advantages to be cheap, and burns quite well. It is not pure, generally used between 75 an 90 percent of volume ratio with water for the rest. That water is used to lower the temperature combustion and to keep the engine cool enough to stay in one piece. Rocket-grade kerosene (RP-1) has been introduced later to replace alcohol, providing a better volume efficiency. | |||
used | |||
( | |||
Alcohol seems to be a very good low cost solution. RP-1 is still used nowadays, and is 20% more efficient than alcohol with a liquid oxygen (LOX) oxidizer. The next question is thus: should we use some pure alcohol, alcohol/water blend or alcohol/something else blend? | |||
=== | '''E85''', a 85 percent alcohol and 15 percent gasoline fuel recently put on the automotive fuel market, makes a promising rocket fuel. Its efficiency should be slightly better than alcohol, still being very cheap, around £0.5 a liter. | ||
Alcohol has good regenerative cooling properties but the non-refined 15% hydrocarbon in it [[RocketEngines#Cooling_for_a_LOX.2FE85_engine|may prevent]] to use it as a coolant. E85 has a different air-fuel ratio than gasoline, requiring less oxygen (or more fuel) to burn, which can be a good thing for us since a cheap LOX tank may be heavy, so the smaller the better. | |||
===Oxidizer=== | |||
''Main page: [[Propellants]].'' | |||
Liquid Oxygen ([[LOX]]) is the obvious/best choice as oxidizer and for high Isp. However, it has the big drawback of being a cryogenic fluid, implying cryogenics [[Rocket_Main_Tank|storage]], cautious manipulation, all that making it quite expensive and complicated. See the [http://books.google.fr/books?id=1OC8zeol7uMC cryogenic engineering book]. | |||
Alternatives are [https://en.wikipedia.org/wiki/Nitrous_oxide#Rocket_motors Nitrous oxide] and [https://en.wikipedia.org/wiki/Hydrogen_peroxide Hydrogen peroxide]. The latter would be better, since it's more dense, but it seems complicated and expensive to have it manufactured at a high concentration. | |||
===Engine=== | ===Engine=== | ||
[ | ''Main page: [[RocketEngines|rocket engines]]'' | ||
The pump is also a major concern, especially for cost and chamber pressure capability. Turbopumps are used on full-scale commercial rockets, but are very complex to build and design. Xcor has created and demonstrated since 2003 a [http://www.xcor.com/products/pumps/ piston pump] for LOX, which is now used on a 1,500 lb-thrust (6.6 kN) LOX/kerosene engine. | |||
[https://en.wikipedia.org/wiki/Aerospike_engine Aerospike] engines may be considered, although they are more efficient than bell shaped nozzles at low altitudes and that we want to launch from high altitude. See web page on [http://www.k-makris.gr/RocketTechnology/Nozzle_Design/nozzle_design.htm nozzle design]. | |||
===Trajectory=== | ===Trajectory=== | ||
The trajectory has to be precise enough to get a launch authorization for | The trajectory has to be precise enough to get a launch authorization for a specific orbit, and in a more practical way, to have orbital parameters matching the mission requirements. Trajectory is closely tied to the [[Flight_at_high_altitude#Approaches_overview|initial release parameters]], the [[Rocket:First_approximations|flight parameters]] such as thrust and aerodynamics, and [https://en.wikipedia.org/wiki/Attitude_control_(spacecraft) attitude control], which depends on sensors and actuators: | ||
a specific orbit. | |||
determine position of the sun and the Earth's horizon. Accelerometers, digital | '''[[EmbeddedRocketComputer#Sensors|sensors]]''': cameras can probably be used on the rocket to determine position of the sun and the Earth's horizon. That will have to be validated, but even if it only allows launches at specific times with clear skies, it can be acceptable for a low-cost launch system. Accelerometers, digital gyroscopes and a compass are really cheap nowadays and can be used for attitude monitoring too. They will likely be used in the fast attitude control loop and to refine the attitude calculated by the camera system. | ||
gyroscopes and a compass are really cheap nowadays and can be used too. | |||
'''Actuators''': if sensors are available, actuators are different story. Two ways of changing attitude of a rocket are generally used, as fins have no impact in the vacuum of space: | |||
* the vector of exhaust gas of the rocket engine can be controlled. This is generally done in modern engines using a gimbal mount and hydraulic actuators, or more in a more innovative way, using electromagnetic actuators like [https://en.wikipedia.org/wiki/Vega_rocket#P80 Vega's P80]. Another solution is to put fins at the output of the engine nozzle. | |||
* control jets (also known as the [https://en.wikipedia.org/wiki/Attitude_control_(spacecraft)#Thrusters RCS]) are used to control the attitude of the rocket. It's often the case for roll control, when the steam generated for turbopumps is not reintroduced in engines but used to control rool, as in SpaceX' Merlin engine. For pitch and yaw, it requires an independent system, generally based on mono-propellant thrusters. | |||
Both solutions pose complicated design issues on the rocket's or engine's hardware, but are mandatory. This is one of the big differences between sub-orbital and orbital space flight. | |||
rocket engine | |||
the | |||
The trajectory itself is a balance between vertical speed, minimizing drag of the rocket that wastes its Delta V capability, an horizontal speed, required to reach orbit. | |||
==The satellite== | ==The satellite== | ||
Lots of strategies for the tracking of the satellite have been exposed: | Lots of strategies for the tracking of the satellite have been exposed: flashing light device, radioactive, EM emitting, mirrors... It's not really the issue for now, and others (like [http://www.wikisat.org/?p=632 WikiSat]) have been working on it already. | ||
flashing device, radioactive, |
Latest revision as of 00:55, 6 November 2017
N-Prize and reflections on low-cost access to space
This Web site aims to gather research in the field of astronautics, rocketry and other technologies that can be used for the N-Prize competition, and more generally, to put microsatellites in orbit at low cost. It is not an official Web site for the N-Prize, the official being here: http://www.n-prize.com/. The goal of this competition is roughly to reproduce the great achievement of the Sputnik in 1957, but for a 20g satellite and with spending less than £1000. However, this Web site and its associated research will not stop after the contest is over, this is more a long term (should I say lifetime?) project. It is hosted by the Open Technology And Science Knowledge Initiative (OTASKI).
I'm not part of a team for the N-Prize, nor did I register one, because I don't really have the expertise and resources to actually build something in time before the deadline of the contest in september 2013. Anyway, if you find this project interesting, join and participate! Maybe if we are enough to work on the project, it is possible to make it in time. It is also possible to provide a part of the challenge and join together with another team providing the other part. Other teams have for example been developing satellites, rocket engines, and so on.
What is the LCAS project?
LCAS, standing for low-cost access to space, aims to provide an open and innovative low-cost orbital launch system for very small satellites (less than 10 kg). Research has led us to consider using an aircraft for rocket launches (air-to-orbit), the body of the plane being the rocket itself. The rocket, or a part of it, as in any other orbital launch system, would achieve orbit and thus could embed a minimum of science, making optional the use of a real satellite as payload. Since the main constraint is to have low costs, we'll have to design and build the carrier plane first, including its turbofan engines, which is probably the hardest part of the whole project, and as far as we know has never been done by amateurs.
We thus currently focus on the turbofan research and design, on which depends everything else. We may then consider helping other N-Prize teams if this is done in time, or other similar projects outside the contest, by providing them those engines and help with aircraft design and rocket integration. Some other parts of the aircraft/rocket are also being studied, for example the software control and the low-cost sensors that can be used to render the aircraft autonomous at first, then make the rocket go into space and reach a controlled orbit.
News
News are also available on twitter @OTASKI
October 2017: A talk was made at SpaceUp about the use of nano-spacecraft as a cheap way to explore the solar system (spoiler: there is no cheap way). See the presentation.
December 2015: CFD is has resumed for a few months. Basic RF telemetry has been validated using a high-altitude balloon, thanks to the resources of ukhas, but for a rocket, a much more important data rate is required. That would either require more directional receiving antennas or a more powerful emitter, which requires an amateur radio license.
Most pages on this wiki are outdated or unfinished and would not be useful for somebody familiar with aerospace. There is one page that can be still useful, about rocket mass estimation. A simple program was developed and some graphs are shown at the bottom, to easily compute and visualise which parameters cause the mass of an air-to-orbit rocket to change.
December 2013: CFD has been stalled for a few months. In parallel, work on RF telemetry has started. First prototype will be tested in a high-altitude balloon in August 2014, in order to validate the RF module and the ground receiver.
March 2013: Since December, learning CFD has been the main activity, and it will probably remain so next months. A page has been created to give CFD beginners some interesting links. CFD has a steep learning curve, but learning how to use it will have huge benefits for the project in the long term:
- evaluate the lift and drag associated with supersonic wings/fins on the aircraft-launched rocket will enable us to refine the rocket mass (File:Rocket mass.c) model
- hopefully have a theoretical validation of our high-altitude turbofan alternative design
- simulate different wing profiles at high-altitude subsonic conditions for the carrier aircraft
- evaluate aircraft and engine capability on lift-off and early flight conditions while they are both tailored for high altitude flight
- refine the heat transfer approximations (File:Heat transfer to rocket tank.c) for cryogenics tank vaporization, used in the rocket mass model for tank dimensioning, with a better climb profile input too.
December 2012: A simple rocket trajectory model has been made in order to evaluate the trajectory of an aircraft- or balloon-released-rocket. It appears that the Delta V taken for granted for gravity drag for these rockets, around 800 m/s, is quite erroneous, or not possible with the expected overall thrust-to-weight ratio. Without taking into account the aerodynamic effects like lift and drag, for a ratio of around 1.5, the Delta V for gravity drag is at least 1300 m/s. To reduce it, the ratio should be higher, like 3.0, in that case it may be possible to have only 800 m/s Delta V for gravity, but the mass of the engine would be much higher. See a solution to this issue.
The program evaluating the mass of the rocket has been refined (v3), and the forgotten pipe linking the upper tank to the engine adds approximately 150 kg of wet mass too (File:Rocket mass.c).
Incoming works will study aerodynamic lift and drag of a simple rocket and of a rocket with small supersonic wings to better evaluate the gravity drag for our mass evaluation.
November 2012: Rocket mass has been estimated to 150 kg. Turbofan engines parameters can now be calculated for a real application: aircraft carrier for air-to-orbit single stage rocket, tailored for pico and nanosatellites.
Also, graphs have been created to illustrate the importance of various rocket design parameters, such as thrust-to-weight ratios, mass ratio, payload mass. The program (File:Rocket mass.c) modelling the mass of rockets is being refined and the cryogenic propellant vaporization has been evaluated by another program (File:Heat transfer to rocket tank.c). Both programs are freely available.
August 2012: A first step in the project realization will be a turbofan's compressor blade manufacturing, in order to validate the manufacturing process suitability and low cost for the turbofan. The first compressor stage prototype has to be designed in this optics. However, that requires having a first approximation of the rocket mass in order to also have an estimation of the aircraft size and mass, from which we can estimate turbofan engine's properties: inlet speed, required thrust, blade length, RPM and so on. Blade manufacturing will mostly rely on a thermocaster that we'll have to design too.
May 21, 2012: Boeing has also announced its low cost orbital launch system, based on the WhiteKnightTwo carrier craft and a hypersonic air-breathing first and second stages.
May 2012: Study of aerodynamics is still heavily under way in order to validate our alternate turbofan mode of operation. This is the first thing to validate before the project can enter a real engine design phase of the engine, which will in turn allow the plane to be designed.
How to escape from Earth?
Rockets have been used for more than 50 years to escape the gravity of earth. They are good for three things: create an important thrust, go fast, and burn a large amount of propellant. Indeed, the efficiency of a propulsion engine is measured with specific impulse (Isp), and for rocket engines, it is quite low. However, their engine is the only engine that provide the sufficient thrust to climb up with large speeds and to tear of Earth's gravity.
Besides altitude, speed is the most important factor when trying to put an object into orbit. Without it, satellites would fall back down on Earth, even if you climb up at 200 miles. Once again, rocket engines, with their high thrust power can achieve sufficient speed (> 8 km/s) before falling back on Earth.
Rocket trajectories generally tend to form a curve nearing the square angle, with the beginning of the flight being orthogonal to Earth and the final direction being parallel to Earth's surface. The reason is that since they achieve ultrasonic speeds very quickly, the dynamic air pressure on their body (mainly the fairing), resulting in drag, becomes quite important. It is more efficient to first escape the low atmosphere, with its 85% of its whole mass below 11km altitude, and then pitch to gain the horizontal speed needed for orbital injection without being slowed down by atmospheric friction.
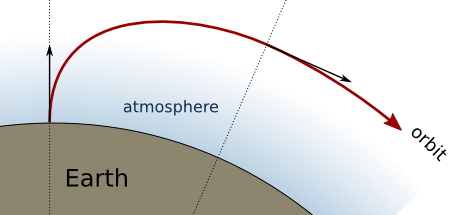
That particular point of the cost of escaping the atmosphere made me thought about using an aircraft to launch a rocket from the upper atmosphere, reducing considerably the air pressure, the drag, and improving trajectory and efficiency. Moreover, the specific impulse of a turbofan is around ten times greater than the Isp of a rocket engine, since it uses oxygen from the atmosphere to burn its fuel, and not some on-board oxidizer. See this article for more information on the differences of rocket and aircraft propulsion efficiency. For the N-Prize, the cost of the aircraft could be deducted from the overall price since if it can be reused.
I started searching and I found out that Orbital already has developped an air-to-orbit launch vehicle, called the Pegasus. It is able to push onto Low Earth Orbit a payload up to 1,000 lbs (450 kg), and it is launched from a full-sized airplane. My goal is thus to study the feasibility of something similar, at very low price, even for the aircraft. A rocket would still be used for air-to-orbit link because nothing else is able to achieve a speed around 9 km/s before falling back on Earth. Some specific technologies can be used to improve efficiency, as explained below in the rocket section.
Several N-Prize teams are working on using Helium or Hydrogen balloons (rockoons) to get to the high atmosphere, up to 35 or 40 km and then launch a rocket. It is a nice solution too, and maybe less expensive in the overall, but balloons are not reusable, suffer from imprecise trajectory due to winds, and provide no initial speed. The initial speed of an aircraft carrier would be quite low too in our first designs, but the potential for a supersonic velocity release is not shut.
Single stage to orbit (SSTO) are also a promising research field for low-cost orbiting. In this video (SpaceX guys), here captured at SpaceUP, they don't even predict the use of attitude control outside the atmosphere to avoid expensive guidance actuators. The main idea of SSTO is that the launch system (rocket) is the payload. It does not even aim to insert a smaller satellite into orbit.
The aircraft
Some aircraft have been exploring the high atmosphere, around 30km high. Contrary to what one would assume, high flight speeds are not needed, if the weight is kept low. The Helios for example, autonomous solar powered aircraft, flights at this altitude at 20km/h. John Powell (JP Aeroospace) is also researching on high altitude propellers and plans to make it to space using a high altitude base for payload transfer to a bigger plane. He describes it well in this video interview. The U-2 is a manned reconnaissance aircraft flying at 21km altitude, cruising at relatively high speeds (690km/h). Those planes are designed with a very long wingspan, and low weight, similar to gliders.
Another kind of design is the fighter jet, for example the MiG-25 which also was an altitude (amongst other) record breaker. It had two powerful turbojet engines with afterburner, allowing him to reach a service altitude of 20km and a maximum altitude of more than 37km. It however required a thrust (200kN) equivalent to the empty weight of the plane and large amounts of fuel to climb this high. The same is true for the SR-71.
These concerns of how high altitude is reached - mainly through high engine power or high lift at subsonic flight - is discussed on the page dedicated to high altitude flight. Currently, the subsonic way is being studied, both for rocket and the aircraft, since a higher release velocity means that the rocket can be smaller, and consequently the plane too.
Nevertheless, we would benefit from speed of the aircraft, speed that wouldn't be needed by the rocket to reach. It is a low speed compared to orbital speed though. Supersonic launch speed would be nice, but very hard to achieve. Currently, only subsonic speed is considered in the project.
The major issue with the aircraft is how to build a £100 turbofan? Small turbofan engines exist, but are made for or by the military, so very expensive, very reliable, and their use is restricted to missiles or UAVs. Small gas turbine engines exist however, even in an axial design, see Bladon Jets or Price Induction for a commercial turbofan for very small jets (nice picture of the fan here).
Links on wing or aircraft design related to speed and altitude: [1].
Staging and recovery
Main page for ground-related aircraft operation and return trip: Aircraft Mission.
Separation from the aircraft is a big concern. If wings and tail are directly mounted on the rocket body and jettisoned, they would not need some guidance or attitude control electronics, just a basic parachute system. If the wings are able to fly without the rocket, two guidance systems are needed: one for the rocket and one to get back the aircraft in one piece for future launches. Keeping the N-Prize in mind, the aircraft part of the space launch system should be reusable, so that it doesn't count in the £1000 limit. In that case, it has to be recovered in good condition, either using a chute and a GPS tracker, or a more complicated autonomous or remotely operated return-to-runway and landing system.
Guidance
Main page: embedded computer.
A satellite navigation system may be used in the plane for position tracking if allowed by their owning entities. Other sensors should be shared with the rocket's embedded computer, if choices made for staging and recovery allow it.
Sun position can be a very good and easy indicator of attitude, as well as earth curve recognition. Video camera is likely to be the main sensor, since it can provide lots of information for very low cost (but for high processing power).
The rocket
Main page: rocket engines, Category page: list of pages.
Some concerns are emphasized in this section, some choices are made too. A list of concerns and how they are handled by existing engine designs can be found on the rocket engines page. For the first approximations of the capabilities and properties of our rocket and rocket engine, for example the minimum weight required to achieve orbit, see the first approximations page.
Fuel
Main page: Propellants.
Propellants represent the most important part of the weight of what we have to launch. It should thus be chosen carefully regarding to its cost, but also regarding their efficiency, the Isp they can produce. This is especially important in an SSTO design.
Alcohol has been used in the early ages of rocketry, in the German V-2 for example. It has the advantages to be cheap, and burns quite well. It is not pure, generally used between 75 an 90 percent of volume ratio with water for the rest. That water is used to lower the temperature combustion and to keep the engine cool enough to stay in one piece. Rocket-grade kerosene (RP-1) has been introduced later to replace alcohol, providing a better volume efficiency.
Alcohol seems to be a very good low cost solution. RP-1 is still used nowadays, and is 20% more efficient than alcohol with a liquid oxygen (LOX) oxidizer. The next question is thus: should we use some pure alcohol, alcohol/water blend or alcohol/something else blend?
E85, a 85 percent alcohol and 15 percent gasoline fuel recently put on the automotive fuel market, makes a promising rocket fuel. Its efficiency should be slightly better than alcohol, still being very cheap, around £0.5 a liter.
Alcohol has good regenerative cooling properties but the non-refined 15% hydrocarbon in it may prevent to use it as a coolant. E85 has a different air-fuel ratio than gasoline, requiring less oxygen (or more fuel) to burn, which can be a good thing for us since a cheap LOX tank may be heavy, so the smaller the better.
Oxidizer
Main page: Propellants.
Liquid Oxygen (LOX) is the obvious/best choice as oxidizer and for high Isp. However, it has the big drawback of being a cryogenic fluid, implying cryogenics storage, cautious manipulation, all that making it quite expensive and complicated. See the cryogenic engineering book.
Alternatives are Nitrous oxide and Hydrogen peroxide. The latter would be better, since it's more dense, but it seems complicated and expensive to have it manufactured at a high concentration.
Engine
Main page: rocket engines
The pump is also a major concern, especially for cost and chamber pressure capability. Turbopumps are used on full-scale commercial rockets, but are very complex to build and design. Xcor has created and demonstrated since 2003 a piston pump for LOX, which is now used on a 1,500 lb-thrust (6.6 kN) LOX/kerosene engine.
Aerospike engines may be considered, although they are more efficient than bell shaped nozzles at low altitudes and that we want to launch from high altitude. See web page on nozzle design.
Trajectory
The trajectory has to be precise enough to get a launch authorization for a specific orbit, and in a more practical way, to have orbital parameters matching the mission requirements. Trajectory is closely tied to the initial release parameters, the flight parameters such as thrust and aerodynamics, and attitude control, which depends on sensors and actuators:
sensors: cameras can probably be used on the rocket to determine position of the sun and the Earth's horizon. That will have to be validated, but even if it only allows launches at specific times with clear skies, it can be acceptable for a low-cost launch system. Accelerometers, digital gyroscopes and a compass are really cheap nowadays and can be used for attitude monitoring too. They will likely be used in the fast attitude control loop and to refine the attitude calculated by the camera system.
Actuators: if sensors are available, actuators are different story. Two ways of changing attitude of a rocket are generally used, as fins have no impact in the vacuum of space:
- the vector of exhaust gas of the rocket engine can be controlled. This is generally done in modern engines using a gimbal mount and hydraulic actuators, or more in a more innovative way, using electromagnetic actuators like Vega's P80. Another solution is to put fins at the output of the engine nozzle.
- control jets (also known as the RCS) are used to control the attitude of the rocket. It's often the case for roll control, when the steam generated for turbopumps is not reintroduced in engines but used to control rool, as in SpaceX' Merlin engine. For pitch and yaw, it requires an independent system, generally based on mono-propellant thrusters.
Both solutions pose complicated design issues on the rocket's or engine's hardware, but are mandatory. This is one of the big differences between sub-orbital and orbital space flight.
The trajectory itself is a balance between vertical speed, minimizing drag of the rocket that wastes its Delta V capability, an horizontal speed, required to reach orbit.
The satellite
Lots of strategies for the tracking of the satellite have been exposed: flashing light device, radioactive, EM emitting, mirrors... It's not really the issue for now, and others (like WikiSat) have been working on it already.